Ультрахолодный газ ферми-атомов и бозе-молекул
Эксперименты с ультрахолодными атомными ферми-газами влияют на дисциплины, лежащие далеко за пределами атомной физики, поскольку к ферми-газу атомов в ряде случаев применимы те же теоретические модели, что и к другим ферми-системам:
- нейтронным звездам и ядрам тяжелых атомов;
- кварк-глюонной плазме, существовавшей во времена Большого взрыва и получаемой сейчас на ускорителях элементарных частиц;
- гелию-3 в жидких фазах;
- будущим комнатнотемпературным сверхпроводникам, создание которых – один из приоритетов физики твердого тела.
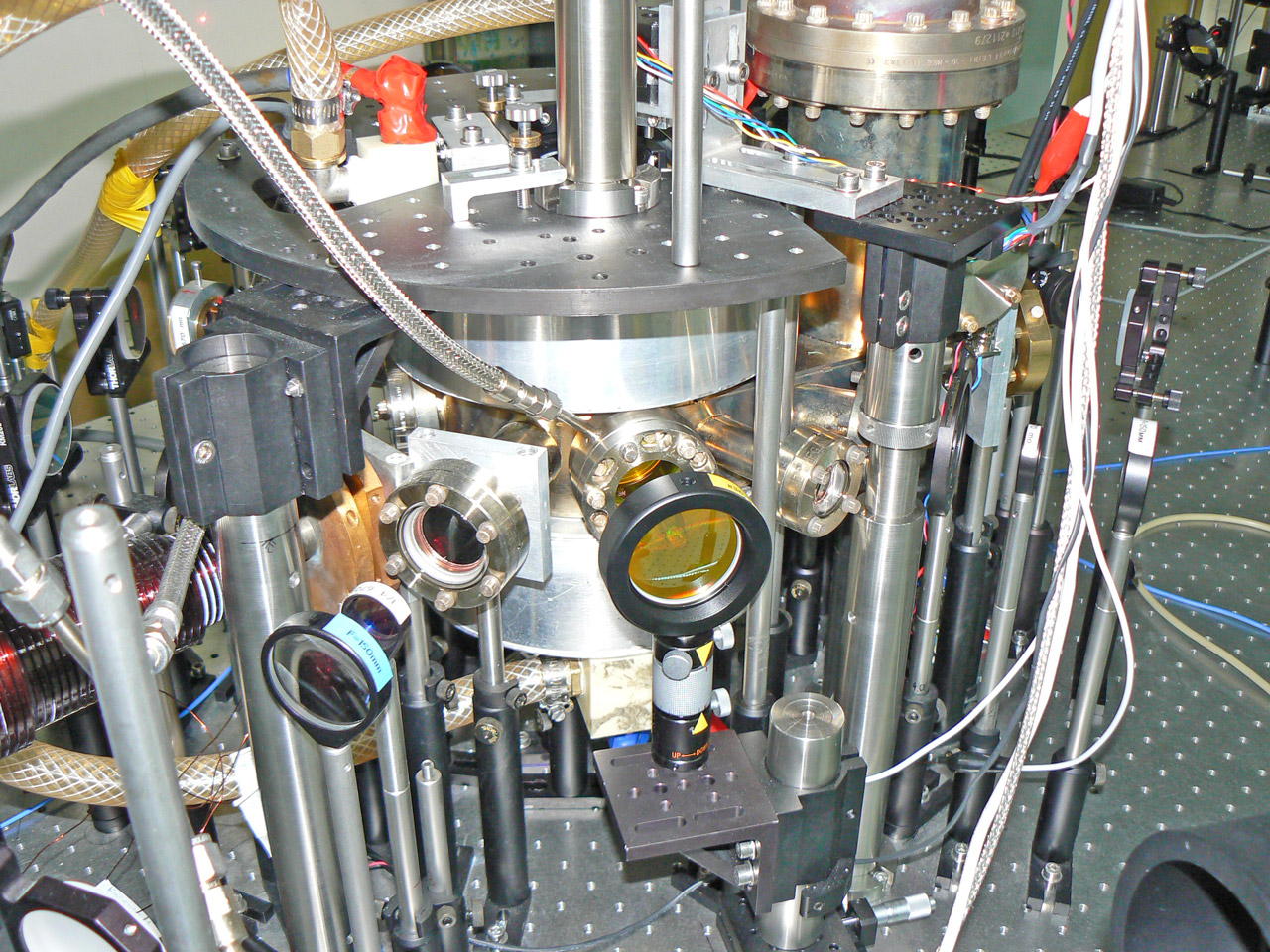
Ультрахолодный атомный газ – уникальный инструмент для проверки этих теоретических моделей, поскольку в атомной системе отсутствуют неконтролируемые дефекты, а параметры могут непрерывно перестраиваться в широких пределах. Перестраиваемые параметры включают в себя взаимодействие, плотность, спиновый состав, температуру, размерность.
В ферми-газе атомов и полученном из него бозе-газе молекул наблюдались фундаментально новые явления: сверхтекучесть с максимально сильным (резонансным) спариванием фермионов; плавный переход от фермиевской статистики к бозевской; когерентные химические реакции, при которых вещество находится в виде суперпозиции начальных и конечного продуктов реакции. Эти эффекты были предсказаны ранее для других систем, но увидеть их удалось лишь в экспериментах с атомными газами при сверхнизких температурах.
Сотрудниками ИПФ РАН (А. В. Турлапов, К. А. Мартьянов, В. Б. Махалов) создана первая в России экспериментальная установка для приготовления квантово-вырожденного газа атомов и молекул как в режиме фермиевского вырождения, так и в режиме бозе-эйнштейновской конденсации. Ультрахолодный газ приготавливается в вакууме методами лазерного пленения и охлаждения. Достигнута наименьшая в стране температура, около 10 нК.
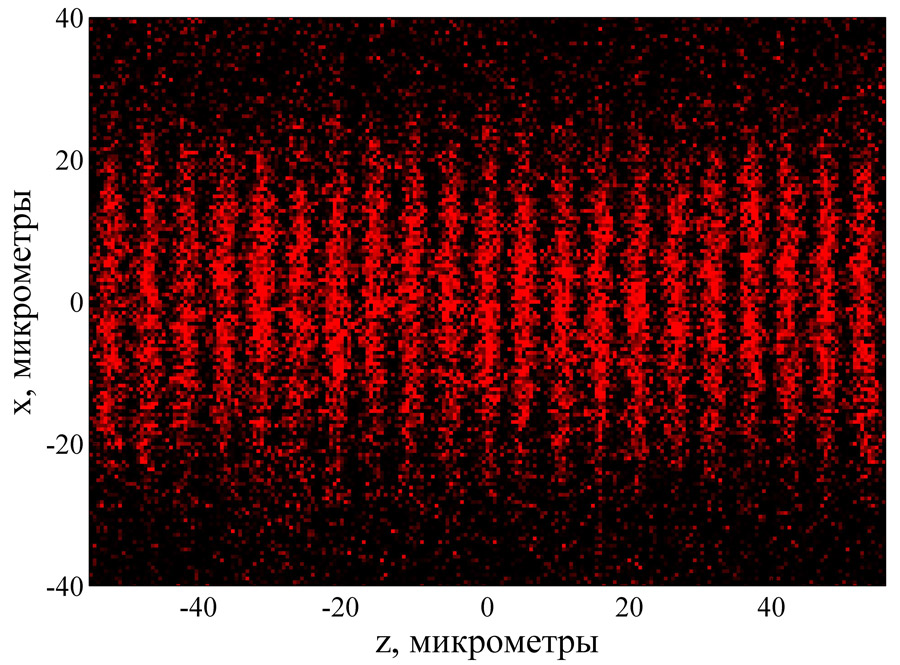
Впервые в мире двумерный газ атомов-фермионов приготовлен в состоянии квантового вырождения в нашем институте. Созданная двумерная ферми-система представляет собой газ атомов лития-6, который удерживается в серии консервативных потенциалов, образованных пучностями стоячей электромагнитной волны ИК-диапазона. Газ, плененный в каждой пучности, является изолированной двумерной системой. Это первый случай, когда распределение фермионов в какой-либо двумерной системе наблюдается напрямую, что дает новые возможности для диагностики.
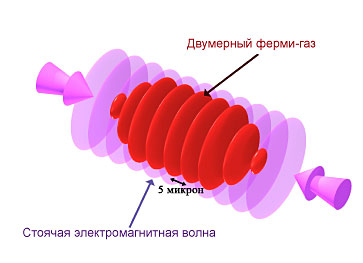
Понижение пространственной размерности кардинально влияет на физику фазовых переходов. Например, в двумерных системах, в отличие от трехмерных, невозможна бозе-эйнштейновская конденсация, хотя сохраняется возможность образования сверхтекучей фазы. Двумерный ферми-газ атомов, приготовленный в ИПФ, может стать платформой для изучения новых явлений. Возможно, впервые удастся наблюдать конденсацию куперовских пар в состояния с ненулевым импульсом. Некоторые возбуждения двумерной системы, возможно, будут фермионами Майораны, для которых частица и античастица совпадают. Сверхтекучесть двумерного ферми-газа может стать экспериментальной моделью высокотемпературной сверхпроводимости и служить для проверки соответствующих теорий, поскольку динамика электронов в современных высокотемпературных сверхпроводниках двумерная.
Методы лазерного охлаждения и пленения вещества, используемые для приготовления ультрахолодных ферми- и бозе-газов атомов и молекул, имеют технологическое применение. Наиболее точные стандарты частоты и времени используют в качестве рабочего вещества газ атомов при температурах от десятков нанокельвин до единиц микрокельвин. Подобные стандарты времени важны для высокоскоростной передачи данных и повышения точности спутниковых навигационных систем.
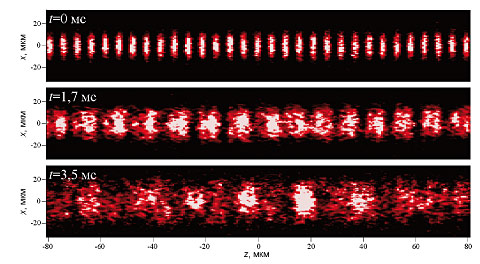